Microelectronics & Quantum Engineering
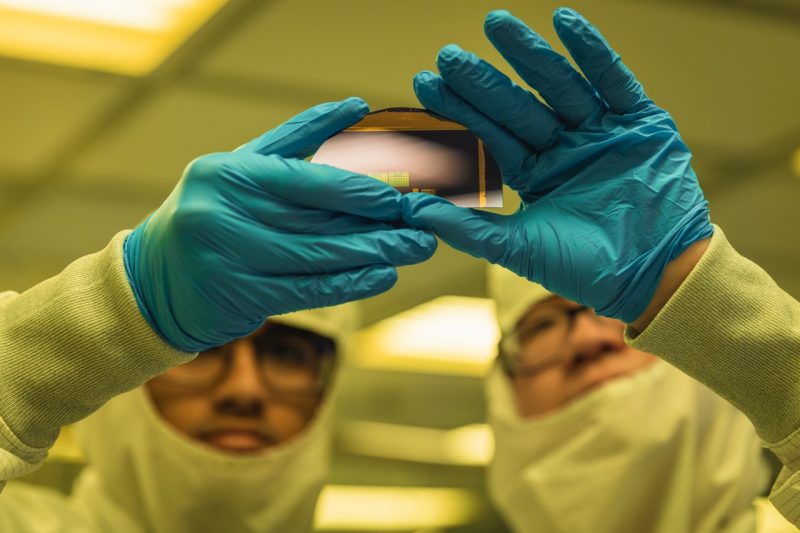
Microelectronics and quantum engineering push the boundaries of scale. Whether it is transforming bulky benchtop equipment into a cell phone sized handheld device, delivering ultra-rapid computation in microprocessors, or storing massive amounts of power in ever shrinking electrical circuits, microelectronics research seeks to develop and miniaturize technologies that will unlock new possibilities. Quantum engineering underpins a massive technological shift over the last century. Revolutionary new sensing, computational, navigational, and communication devices have all been made possible by advances in quantum technology.
Highlighted Research
Inductors and transformers are essential to switch-mode power converters. They are large, heavy, and difficult to integrate in a power converter. Traditional manufacturing often requires pressing, heating, shaping, and winding, which restricts design options. Novel designs that are more efficient (lower loss) and use less magnetic material, such as uniform or constant flux density inductors, are too expensive and take too long to make by traditional means. We have developed a series of magnetic paste materials—both powder-iron and ferrite systems—and used them successfully to make inductors and/or transformers by additive manufacturing. The materials we developed allow for rapid prototyping of novel designs of the magnetic components and ease their integration in power converters.
An ongoing research project involves the design and fabrication of WBG power modules for better thermal dissipation, higher temperature capability, lower parasitics for fast switching, and improved thermo-mechanical reliability.
Current silicon-based CMOS technology is nearing the physical limits of its scaling potential, and increasing data rates pose a challenge to the transmission of electrical signals—while also maintaining low power consumption, low delay, and a high signal-to-noise ratio. Interconnect bottlenecks for inter- and intra-chip communication are projected to be major impediments to energy-efficient performance scaling, thus necessitating optical interconnects com-patible with future CMOS process technologies. Co-integration of electronic and photonic materials and devices with Si manufacturing processes is considered one of the most promising ways to realize the potential of semiconductor compounds. ECE researchers are exploring applications of this integration in optical interconnects, chip-to-chip communication, and low-power and high-speed computing by using cheaper, larger Si wafers. Such monolithic chips will aid and enhance the everyday computing and communication experience for civilian and defense applications.
Resistive switching cell memory, a non-volatile memory based on floating gate MOSFET technology, is a promising technology to supplant current non-volatile memory. In addition to having speed, scalability, density, endurance, and reliability advantages over the current floating gate (FLASH), they do not require silicon substrate. This means they can be stacked in multiple layers in the CMOS metallization, allowing for high densities. They can be placed directly above the logic area, solving the so-called latency problem. ECE researchers are manufacturing the resistive switching devices, and then electrically characterizing them in an effort to understand the basic mechanisms governing their behavior. We are exploring this technology as it ap-plies to memory and neuromorphic applications.
The Microelectromechanical Systems (MEMS) Laboratory at Virginia Tech has been at the forefront of research to develop a field-portable micro gas chromatography system comprising low-power MEMS components including preconcentrators, gas separation columns, and detectors. These systems for on-the-spot analysis of complex gaseous samples, like the air we breathe, can capture analytes even at very low concentrations and then separate and identify the presented compounds through a chromatographic mechanism. We are working on an NSF-funded project to develop Fast Odor Chromatographic Sniffers (FOX-on-chip) that can detect illicit adulteration in food by parallel analysis of the mixture through microfluidic channels and subsequent autonomous data. In line with this project, we are demonstrating the concept of digital chromatography or digital smell. The primary concept is to create electronics signatures (barcodes) for odors (smells) that can be synthesized in a distant location.
Through collaboration with hospitals and bio-medical research communities, we are developing microfluidic channels that can decipher bioelectrical and biomechanical properties of living cells, looking for abnormal cells through alteration in their biophysical signatures. We are analyzing normal and cancerous breast cells, and we find biophysical cues that can be used for early cancer diagnosis or for assessing the efficacy of chemo-therapeutic regimens. We are also investigating the sparsely concentrated circulating tumor cells in the blood of cancer patients through microfluidic constructs with on-chip electrical impedance outputs. Initial results using spiked cancer cells in mouse blood samples have shown the feasibility of our approach.
ECE researchers are developing the next generation of highly robust circuit designs. These circuits, which can withstand rapid changes in voltage and current, utilize specially designed diodes, semiconductors, and transistors to improve system safety, reliability, and durability. This technology has potential for applications in automotive powertrains, power grids, and green energy generation and storage.
Imagine holding the power of a huge benchtop analysis tool in the palm of your hand. ECE researchers are making that dream a reality. By combining technologies such as Microelectromechanical Systems (MEMS), microfluidics, and bioelectrical/biomechanical sensors, these research teams are developing new, portable sensing equipment for applications such as early cancer detection, pharmaceutical quality control, drug efficacy testing, and others. These technologies will allow the lab to be brought into the field for use in forensics, emergency medicine, or national security.
ECE researchers are developing new lab-on-achip style sensors that harness the capabilities of quantum information processing to deliver acousto-optical and electro-optical sensing on a nano scale. In other labs, the same small scale approach is being used to develop the next generation of oscillators and navigational tools. These technologies rely on a combination of 3D nanofabrication, unique sensor design and optimization, and efficient computation.
Other Research Topics
- Power electronic packaging and materials
- Packaging of power semiconductor devices and modules
- Material synthesis for die bonding, electrical insulation, and magnetic components
- Reliability of power semiconductor packages
- Wide-bandgap power semiconductors modules